Welcome
to the dissolved oxygen (D.O.) theory Page. Dissolved oxygen
from A to
Z.
Dissolved Oxygen:
The
air we breathe contains about 20% oxygen. Fish and other aquatic organisms
require oxygen as well. The term Dissolved Oxygen
(DO or D.O.) refers to the
amount of free oxygen dissolved in water which is readily available to
respiring aquatic organisms. State water quality standards often express
minimum concentrations of dissolved oxygen which must be maintained in
order to support life as well as be of beneficial use. Levels of dissolved
oxygen below 4-5 milligrams per liter affect fish health and levels below
2 milligrams per liter can be lethal to fish.
Additionally,
biochemical oxygen demand (BOD) is commonly used with reference to
effluent discharges and is a common, environmental procedure for
determining the extent to which oxygen within a sample can support
microbial life. The test for BOD is especially important in waste water
treatment, food manufacturing, and filtration facilities where the
concentration is crucial to the overall process and end products. High
concentrations of DO predict that oxygen uptake by microorganisms is low
along with the required break down of nutrient sources in the medium.
Basic
principles of Polagrography cell:
Liquid
and Air state of equilibrium is reached when the partial pressure of
oxygen, i.e. the part of the total pressure that is due to oxygen, is
equal in air and in liquid. The liquid is then saturated with oxygen.
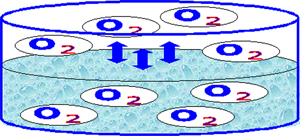
Figure
1.1 Air and liquid oxygen equilibrium

Polargram:
When
an electrode of noble metal such as platinum or gold is made 0.6 to 0.8 V
negative with respect to a suitable reference electrode such as AgAgCl or
an calomel electrode in a neutral KCI solution (see Figure 1.2), the
oxygen dissolved in the liquid is reduce at the surface of the noble
metal.
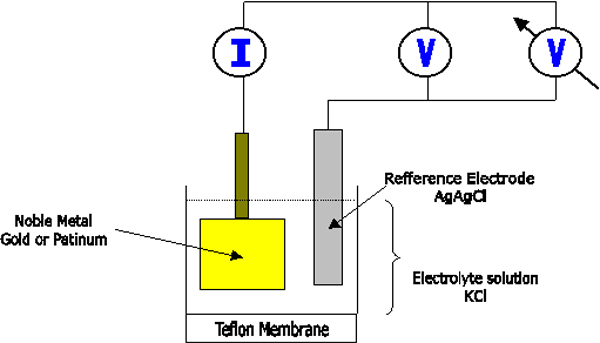
Figure
1.2 Polarographhy diagram
This
above phenomenon can be observed from a current to voltage diagram called a
polarogram of the electrode. As shown in Figure 1.3a, the negative voltage
applied to the noble metal electrode (called the cathode) is increased,
the current increases initially but soon it becomes saturated. In this
plateau region of the polarogram, the reaction of oxygen at the cathode is
so fast that the rate of reaction is limited by the diffusion of oxygen to
the cathode surface. When the negative bias voltage is further increased,
the current output of the electrode increases rapidly due to other
reactions, mainly, the reduction of water to hydrogen. If a fixed voltage
in the plateau region (for example, - 0.6V) is applied to the cathode, the
current output of the electrode can be linearly calibrated to the dissolved oxygen
(Figure 1.3b). It has to be noted that the current is
proportional not to the actual concentration but to the activity or
equivalent partial pressure of dissolved oxygen, which is often referred
to as oxygen tension. A fixed voltage between -0.6 and -0.8 V is usually
selected as the polarization voltage when using Ag/AgCl as the reference
electrode or any other EID's dissolved oxygen electrodes.
Additionally
for physical and chemical correctness, partial pressure in a liquid
actually refers to the fugacity. In the pressure range relevant to the
measurements at hand, it is acceptable to equate the two values and this
allows us to restrict the following considerations to the partial
pressure. In dry, atmospheric air, the partial pressure of oxygen is
20.95% of the air pressure. This value is reduced over a water surface
because water vapor has its own vapor pressure and a corresponding partial
pressure.
Figure
1.3 (a) Current to voltage diagram at different oxygen tension; (b)
Calibration obtained at a fixed polarization voltage of –600 mV.
When
the cathode, the reference electrode, and the electrolyte are separated
from the measurement medium by a polymer membrane, which is permeable to
the dissolved gas but not to most of the ions and other species, and when
most of the mass transfer resistance is confined in the membrane, EID’s
electrode system can measure oxygen tension in various liquids. This is
the basic operating principle of the membrane covered polarographic
Dissolve oxygen probe (Figure 1.4).
The
basic principle underlying the electrochemical determination of oxygen
concentration is the use of membrane covered electrochemical sensors. The
main components of the sensors are the oxygen permeable membrane, the
working electrode, the electrolyte solution and a
possible reference electrode. A voltage is applied between the gold
(platinum or silver) cathode and the anode that consists of either lead or
silver (AgAgCl), and causes
the oxygen to react electrochemically. The higher the oxygen concentration
the higher the resulting electric current. The current in the sensor is
measured and, after calibration, converted into the concentration of dissolved oxygen.
If the anode is made of
silver, the meter applies the required voltage (polarographic sensor). If
it is made of lead, the sensor is self-polarizing, i.e. the voltage is
generated in the sensor by the electrodes themselves, comparable to the
process in a battery (galvanic sensor). The meter merely evaluates the
current.
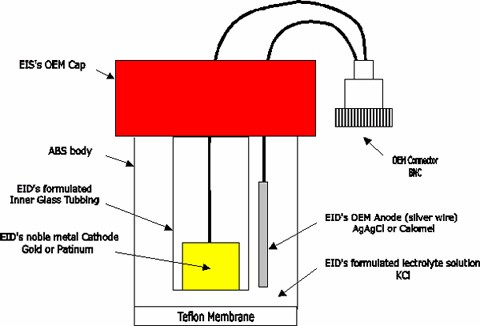
Figure
1.4 Basic Polarographhy electrode

EID’s
polaragraphoc dissolved oxygen electrode picture:
EID’s
ELECTRODER - ABS
body Dissolved Oxygen Sensor (ADO)
EID’s
dissolved oxygen, Probe, polaragraphic, ABS body, 12mm * 120mm, with 10K
Negative Temperature Compensation
Figure
1.5 Basic Polarographhy-electrode

Electrode
reactions:
For
our polarographic electrodes, the reaction proceeds as follows:
H202
+ 2e- -> 20H-
The
reaction tends to produce alkalinity in the medium together with a small
amount of hydrogen peroxide.

Number
of electrons involved:
Two
principal pathways were proposed for the reduction of oxygen at the noble
metal surface. One is a 4-electron pathway where the oxygen in the bulk
diffuses to the surface of the cathode and is converted to H2O
via H2O2 (path a in Fig. 1.6). The
other is a 2-electron pathway where the intermediate H2O2 diffuses directly out of the
cathode surface into the bulk liquid (path b in Figure 1.6). The oxygen
reduction path may change depending on the surface condition of the noble
metal. This is probably the cause for time-dependent current drift of
polarographic sensors. Since the hydroxyl ions are constantly being
substituted for chloride ions as the reaction starts, KCI or NaCl has to
be used as the electrolyte. When the electrolyte is depleted of Cl-,
it has to be replenished.
2e-
2e- à (a) à H2O2
O2 à
O2 à
H2O2
Diffusion
à (b) à H2O2
Figure
1.6 alternative pathway of oxygen reduction at cathode surface

Calibration:
Calibration
must be carried out for dissolved oxygen measurements on a regular base.
This is because the measuring process consumes the electrolyte solution in
the sensor head, as shown by the electrode reactions presented above. The
ions of the electrolyte solution bind the released metal ions, thereby
changing the composition of the solution. The recommended calibration
period depends on the oxygen sensor used and ranges from one week for
pocket instruments to 1-2 months.
Each
linear calibration function is defined by at least two points. For dissolved oxygen
measurements with EID meter and/or logger, one of the
points on the line is the zero point of the sensor. At the zero point, the
sensor signal obtained in the absence of oxygen lies below the resolution
of the sensor. This point is called the zero-current point of the sensor.
The second point of the calibration line can be set as required. Its
position is based on the fact that, in a state of equilibrium, the partial
pressure of oxygen in liquid and air is equal.
Figure
1.4b Two-point calibration
The
rate at which oxygen enters a dissolved oxygen probe is a function of:
As
described above calibration routines for dissolved oxygen probes use a two
point linear calibration where one point is at zero mg/L oxygen and the
second point is at saturation or equilibrium with the atmosphere, C*
. The
zero measurement is not zero volts due to the conductivity of the
electrolyte between the electrodes as well as any errors in the analog
signal conditioning circuit. For the circuit and probe system used in the
Environmental lab the zero measurement
is approximately 1 mV (where approximately 200 mV corresponds to
saturation levels of oxygen) and hence the zero measurement is not
significant. Thus a single point calibration is used.
C*
is a function of the atmospheric pressure and temperature. The functional
relationship with temperature is implemented using a lookup table (based
on equilibrium at atmospheric pressure) with interpolation. The effect of
atmospheric pressure is implemented as shown (Equation 1 below).
P
C*
=------- f (T)
Patm
The
permeability of the membrane increases about 5% per C degree. I chose to
use 25 C as the reference temperature and thus Kmembrane(Tref)
has a value of 1. The following (equation 2) creates a coefficient
that describes this variation.
Kmembrane(T)=Kmembrane(Tref)e0.05(T-Tref)
The
slope of the linear fit (k) can be calculated after the voltage
corresponding to saturation oxygen is measured (Equation 3 below).
C*cal Kmembrane
C
=------------------
V*cal
The
slope coefficient is placed in the polynomial array.
The
equation for the dissolved oxygen concentration illustrates that the
predicted concentration is a function of sample temperature because Kmembrane
varies with temperature. The coefficient, k, should be independent
of temperature but will vary as a membrane fouls (Equation
4 below)
KV
C=-----------
Kmembrane
Pressure
The
constituents of air have been well defined, and it is known that air
contains 20.946% oxygen. Since the total pressure in the air is the sum of
all of the partial pressures (Dalton’s Law), an atmospheric pressure of
760 millimeters Mercury (mmHg) in dry air will contain a partial pressure
of oxygen (pO2) of approximately 159 mmHg (760 mmHg * 0.20946).
Changes in atmospheric pressure will cause a directly proportional
change in the partial pressure of oxygen in the air.
Atmospheric pressures will vary depending upon altitude and local
weather conditions. Some
average pressures for varying altitudes are listed in Table 1 bellow.
The
relationship between oxygen partial pressure and total atmospheric
pressure should be understood and incorporated into the air calibration in
order to minimize calibration error, which could be as high as 5-10%
dependent upon altitude and local weather conditions.
Most dissolved oxygen meters that have any sort of advanced air
calibration (such as temperature compensation, which will be discussed in
a later section) will be based upon an atmospheric pressure of 760 mmHg.
Most tables of oxygen solubility are referenced to this value.
Because of the change in oxygen partial pressure with changes in
atmospheric pressure, a correction must be made when the pressure varies
from this value. A simple
means of incorporating pressure changes is listed in the “correction
factor” shown in Table 1 bellow. The
value listed is a rough multiplier, which can be used once the initial
oxygen concentration is determined based upon temperature and relative
humidity. A more accurate
calculation for incorporating pressure will be discussed after relative
humidity and temperature effects are investigated.
Some
EID’s dissolved oxygen meters contain a pressure sensing device which
provides compensation for pressure effects when an air calibration is
performed. If you use our
electrode on not-EID-meter, since most meters do not have this, it is
usually necessary to note the average pressure in the local vicinity of
the probe, which will be mostly altitude-based, and adjust the calibration
using the simple correction factor or the more complex calculation
performed later. A mercury
barometer located in the immediate vicinity of the meter will give a
relatively accurate measurement of the local atmospheric pressure if an
older meter with no pressure sensor is used.
Altitude
(ft)
|
Pressure
(mm
Hg)
|
Correction
Calibration
Correction
Factor
|
-540
|
775
|
1.02
|
Sea
Level
|
760
|
1
|
500
|
746
|
0.98
|
1000
|
732
|
0.96
|
1500
|
720
|
0.95
|
2000
|
707
|
0.93
|
2500
|
694
|
0.91
|
3000
|
681
|
0.9
|
3500
|
668
|
0.88
|
4000
|
656
|
0.86
|
4500
|
644
|
0.85
|
5000
|
632
|
0.83
|
5500
|
621
|
0.82
|
6000
|
609
|
0.8
|
Table
1: Oxygen Value Corrected for Pressure (25 °C)
Relative
Humidity and temperature effect and Temperature compensation
If
desired the Eid's probes can be coupled with a temperature thermistor (10K
Ohms) to achieve temperature compensation since Kmembrane
varies with temperature.
The
discussion of pressure effects were based upon atmospheric pressure with
dry air (no moisture content). Whenever
air contains a certain amount of moisture, the atmospheric pressure
contains another source of partial pressure -- water vapor.
If a comparison of the oxygen partial pressure in air with 100%
relative humidity and air with 0% relative humidity is done while both are
at the same atmospheric pressure, the air with 100% relative humidity will
have a lower oxygen partial pressure due to the presence of the water
vapor pressure (pH2O). Water
vapor pressure in air varies with temperature, and is well defined.
The effect of temperature on oxygen partial pressure in moist air
is such that higher temperatures yield lower oxygen partial pressure,
while lower temperatures yield higher pressures.
Note that the effects of relative humidity and temperature can
cause errors when air calibration is performed in dry air, since most of
the current tables and meter temperature compensations are based on air
containing 100% relative humidity. Table
2 bellow shows both the oxygen concentration, which is linear with the
partial pressure of oxygen, that
would be present at 100% relative humidity and 0% relative humidity.
The values only differ by a few percent in ambient air conditions,
and thus is generally ignored. Most dissolved oxygen meters have
temperature compensation for air at 100% relative humidity, and no manual
correction is necessary. However,
many older meters do not have temperature compensation included, and
therefore this calculation must be done manually.
If temperature is not compensated for in the calibration, the error
can be as much as 20 to 30 % for every 10 degrees difference from 25 °C,
and therefore temperature compensation is standard on most dissolved
oxygen meters today. Since
the effects of relative humidity is minimal at all but the highest
temperatures, no current dissolved oxygen meters incorporate any kind of
relative humidity sensing device.
In
order to ensure an accurate temperature and current reading, the probe
must be exposed to the air for enough time to allow thermal equilibrium to
occur. There are often
significant temperature differences between the process water and the
ambient air. Larger
temperature gradients between the two necessitate additional time for
thermal equilibrium to take place. For
instance, a 20 °C
difference between ambient air and process water can cause a calibration
delay of about 30 minutes in many probes for the probe to fully
equilibrate to ambient temperature. Since
most temperature gradients will not be this large, allowing approximately
15 minutes is usually a safe assumption.
It is common for users to calibrate the unit before the dissolved
oxygen meter is reading the stabilized temperature and current value,
which can cause significant error since a difference of even 5 °C
from actual can cause the reading be off by 5 to 10%.
It is often useful to have a calibrated temperature sensor,
accurate to 1 °C
or better, at the calibration location to know when the probe temperature
is reading the correct ambient air temperature.
It
is useful to have an equation which can be used to determine oxygen
concentrations in air based upon temperature, relative humidity, and
pressure. Since the full equation is quite lengthy and complex, two easier
versions are presented to the user, along with Table 2 bellow, to
determine the correct oxygen concentration in air.
Equation 5 bellow should be used with air with 100% relative
humidity, and Equation 6 should be used for air with 0% relative humidity.
Equation
5 (100% Relative Humidity): OS =
(OS’) * (P - p) / (760 - p)
where:
OS
= Oxygen solubility at barometric pressure of interest
OS’
= Oxygen in saturation at one atmosphere (760 mmHg) at a given temperature
P
= Barometric pressure of interest
p
= Vapor pressure of water at the temperature of interest
Example
1:
The
user wishes to calibrate a dissolved oxygen probe in air at an altitude of
3500 feet. The temperature is
30 °C,
and the relative humidity is 100%.
At
an altitude of 3500 feet, the atmosphere pressure will usually be about
668 mmHg (Table 1 above). The
sample temperature is 30 °C,
and the relative humidity is 100%. From
water vapor pressure tables, the water vapor pressure at 30 °C
is 31.8 mmHg. The oxygen saturation
level at 760 mmHg and 30 °C
is 7.54 ppm (Table 2 bellow). Substituting
these values in the above (equation 5) gives the following:
OS
= (7.54)
* (668 - 31.8) / (760 - 31.8) =
6.59 ppm
Example
2:
Assume
the same conditions as in example 1, but with a relative humidity of 0%.
In this case, the value used for the oxygen saturation level would
be 7.87 (Table 2 bellow), not 7.54. The
calculation will change since there will be no water vapor pressure.
Equation
6 (0% Relative Humidity): OS =
(OS’) * (P) / (760 mmHg)
Substituting
the above values into the equation yields the following:
OS
= 7.87 * (668) / (760) = 6.92 ppm
Note:
that the multiplier of (668) / (760) is actually the simplified correction
factor listed in Table 1 above for an altitude of 3500 feet (0.88). Table
3 bellow lists calibration values for varying temperatures pressures at
relative humidity levels of 100%.
Temperature
(Celsius)
|
DO (100% R.H.)
(ppm, mg/L)
|
DO (0% R.H.)
(ppm, mg/L)
|
0
|
14.6
|
14.66
|
1
|
14.19
|
14.26
|
2
|
13.81
|
13.89
|
3
|
13.44
|
13.53
|
4
|
13.09
|
13.18
|
5
|
12.75
|
12.85
|
6
|
12.43
|
12.54
|
7
|
12.12
|
12.23
|
8
|
11.83
|
11.94
|
9
|
11.55
|
11.66
|
10
|
11.27
|
11.4
|
11
|
11.01
|
11.14
|
12
|
10.76
|
10.9
|
13
|
10.52
|
10.66
|
14
|
10.29
|
10.44
|
15
|
10.07
|
10.22
|
16
|
9.85
|
10.01
|
17
|
9.65
|
9.82
|
18
|
9.45
|
9.63
|
19
|
9.26
|
9.45
|
20
|
9.07
|
9.27
|
21
|
8.9
|
9.11
|
22
|
8.72
|
8.95
|
23
|
8.56
|
8.8
|
24
|
8.4
|
8.65
|
25
|
8.24
|
8.51
|
26
|
8.09
|
8.37
|
27
|
7.95
|
8.24
|
28
|
7.81
|
8.12
|
29
|
7.67
|
8
|
30
|
7.54
|
7.88
|
31
|
7.41
|
7.77
|
32
|
7.28
|
7.66
|
33
|
7.16
|
7.56
|
34
|
7.05
|
7.46
|
35
|
6.93
|
7.37
|
36
|
6.82
|
7.27
|
37
|
6.71
|
7.18
|
38
|
6.61
|
7.1
|
39
|
6.51
|
7.01
|
40
|
6.41
|
6.93
|
41
|
6.31
|
6.85
|
42
|
6.22
|
6.78
|
43
|
6.13
|
6.7
|
44
|
6.04
|
6.63
|
45
|
5.95
|
6.56
|
46
|
5.86
|
6.49
|
47
|
5.78
|
6.43
|
48
|
5.7
|
6.36
|
49
|
5.62
|
6.3
|
50
|
5.54
|
6.24
|
Table
2 above: Dissolved Oxygen Solubility vs. Temperature
Temperature
(Celsius)
|
790
|
775
|
760
|
745
|
730
|
715
|
700
|
685
|
670
|
665
|
0
|
15.2
|
14.9
|
14.6
|
14.3
|
14
|
13.7
|
13.4
|
13.2
|
12.9
|
12.6
|
1
|
14.8
|
14.5
|
14.2
|
13.9
|
13.6
|
13.3
|
13.1
|
12.8
|
12.5
|
12.2
|
2
|
14.4
|
14.1
|
13.8
|
13.5
|
13.3
|
13
|
12.7
|
12.4
|
12.2
|
11.9
|
3
|
14
|
13.7
|
13.4
|
13.2
|
12.9
|
12.6
|
12.4
|
12.1
|
11.8
|
11.6
|
4
|
13.6
|
13.4
|
13.1
|
12.8
|
12.6
|
12.3
|
12.1
|
11.8
|
11.5
|
11.3
|
5
|
13.3
|
13
|
12.8
|
12.5
|
12.2
|
12
|
11.7
|
11.5
|
11.2
|
11
|
6
|
12.9
|
12.7
|
12.4
|
12.2
|
11.9
|
11.7
|
11.4
|
11.2
|
10.9
|
10.7
|
7
|
12.6
|
12.4
|
12.1
|
11.9
|
11.6
|
11.4
|
11.2
|
10.9
|
10.7
|
10.4
|
8
|
12.3
|
12.1
|
11.8
|
11.6
|
11.4
|
11.1
|
10.9
|
10.7
|
10.4
|
10.2
|
9
|
12
|
11.8
|
11.6
|
11.3
|
11.1
|
10.9
|
10.6
|
10.4
|
10.2
|
9.94
|
10
|
11.7
|
11.5
|
11.3
|
11
|
10.8
|
10.6
|
10.4
|
10.1
|
9.92
|
9.69
|
11
|
11.5
|
11.2
|
11
|
10.8
|
10.6
|
10.4
|
10.1
|
9.91
|
9.69
|
9.47
|
12
|
11.2
|
11
|
10.8
|
10.5
|
10.3
|
10.1
|
9.9
|
9.68
|
9.47
|
9.25
|
13
|
10.9
|
10.7
|
10.5
|
10.3
|
10.1
|
9.89
|
9.68
|
9.47
|
9.26
|
9.04
|
14
|
10.7
|
10.5
|
10.3
|
10.1
|
9.88
|
9.67
|
9.46
|
9.26
|
9.05
|
8.85
|
15
|
10.5
|
10.3
|
10.1
|
9.87
|
9.67
|
9.46
|
9.26
|
9.06
|
8.86
|
8.65
|
16
|
10.3
|
10.1
|
9.85
|
9.65
|
9.45
|
9.26
|
9.06
|
8.86
|
8.66
|
8.46
|
17
|
10
|
9.84
|
9.65
|
9.46
|
9.26
|
9.07
|
8.87
|
8.68
|
8.48
|
8.29
|
18
|
9.83
|
9.64
|
9.45
|
9.26
|
9.07
|
8.88
|
8.69
|
8.5
|
8.31
|
8.12
|
19
|
9.63
|
9.45
|
9.26
|
9.07
|
8.89
|
8.7
|
8.51
|
8.33
|
8.14
|
7.95
|
20
|
9.44
|
9.25
|
9.07
|
8.89
|
8.7
|
8.52
|
8.34
|
8.15
|
7.97
|
7.79
|
21
|
9.26
|
9.08
|
8.9
|
8.72
|
8.54
|
8.36
|
8.18
|
8
|
7.82
|
7.64
|
22
|
9.07
|
8.9
|
8.72
|
8.54
|
8.37
|
8.19
|
8.01
|
7.84
|
7.66
|
7.48
|
23
|
8.91
|
8.73
|
8.56
|
8.39
|
8.21
|
8.04
|
7.86
|
7.69
|
7.52
|
7.34
|
24
|
8.74
|
8.57
|
8.4
|
8.23
|
8.06
|
7.89
|
7.72
|
7.55
|
7.38
|
7.2
|
25
|
8.58
|
8.41
|
8.24
|
8.07
|
7.9
|
7.74
|
7.57
|
7.4
|
7.23
|
7.06
|
26
|
8.42
|
8.26
|
8.09
|
7.92
|
7.76
|
7.59
|
7.43
|
7.26
|
7.1
|
6.93
|
27
|
8.28
|
8.11
|
7.95
|
7.79
|
7.62
|
7.46
|
7.3
|
7.14
|
6.97
|
6.81
|
28
|
8.13
|
7.97
|
7.81
|
7.65
|
7.49
|
7.33
|
7.17
|
7.01
|
6.85
|
6.69
|
29
|
7.99
|
7.83
|
7.67
|
7.51
|
7.35
|
7.2
|
7.04
|
6.88
|
6.72
|
6.57
|
30
|
7.85
|
7.7
|
7.54
|
7.38
|
7.23
|
7.07
|
6.92
|
6.76
|
6.61
|
6.45
|
31
|
7.72
|
7.56
|
7.41
|
7.26
|
7.1
|
6.95
|
6.8
|
6.64
|
6.49
|
6.34
|
32
|
7.58
|
7.43
|
7.28
|
7.13
|
6.98
|
6.83
|
6.68
|
6.53
|
6.38
|
6.22
|
33
|
7.46
|
7.31
|
7.16
|
7.01
|
6.86
|
6.71
|
6.57
|
6.42
|
6.27
|
6.12
|
34
|
7.34
|
7.2
|
7.05
|
6.9
|
6.76
|
6.61
|
6.46
|
6.32
|
6.17
|
6.02
|
35
|
7.22
|
7.07
|
6.93
|
6.79
|
6.64
|
6.5
|
6.35
|
6.21
|
6.06
|
5.92
|
36
|
7.11
|
6.96
|
6.82
|
6.68
|
6.53
|
6.39
|
6.25
|
6.11
|
5.96
|
5.82
|
37
|
6.99
|
6.85
|
6.71
|
6.57
|
6.43
|
6.29
|
6.15
|
6
|
5.86
|
5.72
|
38
|
6.89
|
6.75
|
6.61
|
6.47
|
6.33
|
6.19
|
6.05
|
5.91
|
5.77
|
5.63
|
39
|
6.79
|
6.65
|
6.51
|
6.37
|
6.23
|
6.1
|
5.96
|
5.82
|
5.68
|
5.54
|
40
|
6.68
|
6.55
|
6.41
|
6.27
|
6.14
|
6
|
5.86
|
5.73
|
5.59
|
5.45
|
41
|
6.58
|
6.44
|
6.31
|
6.18
|
6.04
|
5.91
|
5.77
|
5.64
|
5.5
|
5.3
7
|
42
|
6.49
|
6.35
|
6.22
|
6.09
|
5.95
|
5.82
|
5.69
|
5.55
|
5.42
|
5.28
|
43
|
6.39
|
6.26
|
6.13
|
6
|
5.87
|
5.73
|
5.6
|
5.47
|
5.34
|
5.2
|
44
|
6.3
|
6.17
|
6.04
|
5.91
|
5.78
|
5.65
|
5.52
|
5.39
|
5.25
|
5.12
|
45
|
6.21
|
6.08
|
5.95
|
5.82
|
5.69
|
5.56
|
5.43
|
5.3
|
5.17
|
5.04
|
46
|
6.12
|
5.99
|
5.86
|
5.73
|
5.6
|
5.47
|
5.35
|
5.22
|
5.09
|
4
.96
|
47
|
6.03
|
5.91
|
5.78
|
5.65
|
5.53
|
5.4
|
5.27
|
5.14
|
5.02
|
4.89
|
48
|
5.95
|
5.83
|
5.7
|
5.57
|
5.45
|
5.32
|
5.19
|
5.07
|
4.94
|
4.82
|
49
|
5.87
|
5.75
|
5.62
|
5.49
|
5.37
|
5.24
|
5.12
|
4.99
|
4.87
|
4.74
|
50
|
5.79
|
5.66
|
5.54
|
5.42
|
5.29
|
5.17
|
5.04
|
4.92
|
4.79
|
4.67
|
Table
3 above: Oxygen concentration (ppm) for varying pressures (mmHg) and
temperatures (degrees Celsius) at 100% relative humidity

Calibration
in air saturated with water vapor:
This
requirement is met over large water surfaces, such as lakes or the sludge
activation basin of a wastewater treatment plant.
Note:
EID’s offers special air calibration vessels for laboratory
measurements.

Calibration
in air saturated water:
The
water is aerated until the partial pressure of the oxygen in the water is
the same as in the air. This method is accompanied by some inherent risks:
-
The
air pressure in the aeration tube is always somewhat higher than the
normal air pressure and, therefore, the water is always somewhat
supersaturated after aeration.
-
The
water temperature falls during aeration (latent heat!)
-
If
the experimenter waits until the temperatures are equal, the water
will be somewhat supersaturated.
-
The
point at which the water is completely saturated is difficult to
estimate. There is a risk of under saturation.
-
Oxygen
depleting substances lead to under saturation.
As
you can see from the above list, is clearly preferable to calibration in
air-saturated water.

Checking
the sensor function:
Relative
slope limitation, to evaluate the sensor function despite it, there are
three characteristic measuring points in addition to a visual test.
In
the visual test, the gold cathode is examined visually. If it has lost its
gold or platinum color and is coated with lead or silver, the sensor will
yield values that are too high and will generally no longer be
zero-current-free. This can be corrected by regenerating the oxygen sensor
as described in the operating manual. The gold cathode may only be
polished with a moist special abrasive film using a circular motion with
little pressure. It is imperative that only this special film be used
since a scratched and unpolished electrode surface can harm the sensor and
impair its accuracy.
Attention:
Anodes of lead or silver cannot be polished at all.
One
subjective, visual examination of the sensor can be supplemented by a more
comprehensive test, an evaluation at three specific measuring points: in
air saturated with water vapor (1), in air saturated water (2) and in
oxygen free water (3).
1.
Test in air saturated with water vapor:
The
sensor should obtain a reading between 100 and 104% oxygen saturation in
water-saturated air. If the values lie above this range, the membrane was
probably wet during calibration; perhaps there is too much water in the
calibration vessel. A value above 100% saturation is due to the differing
viscosity of water and air as well as to the surface tension of water. To
put it simply, it is easier for oxygen molecules in the air to permeate
the membrane than for those in the water to dissolved oxygen so. In the measuring mode,
which is the mode in which the test takes place, calculations are based on
a liquid sample and this results in a saturation level over 100%.
2.
Test in air saturated water:
After
calibration, the value in air-saturated water should lie between 97 and
102% saturation. The theoretical value is 100% but is difficult to
reproduce. This relatively large tolerance is due not to the sensor but to
the saturation procedure. This is also the reason why EID successfully
sought an alternative to the conventional calibration procedure in
air-saturated water. If the sensor dissolved oxygen not display a reading within this
tolerance range, it should be sent back to the manufacturer for tests.
3.
Test using zero solution:
This
is to test the zero current point of the sensor. When the oxygen content
is 0 mg, the maximum reading of the sensor should not exceed the
resolution of the meter (1 digit). This test is carried out using sodium
sulfite solution. Sulfite reacts with the dissolved oxygen to form
sulfate, binding the oxygen dissolved in the water.
Preparation
of the solution: Dissolve a teaspoon of sodium sulfite in
100 ml tap water. The solution will be oxygen free after it has stood
undisturbed for 15 minutes. It must remain undisturbed to prevent oxygen
in the surrounding air from re entering the solution. One minute after
submerging paleographic sensors (EID-E-ADO–A001,
EID-E-ADO-A002, etc.)
Into the solution, the meter should display a maximum reading of 2%; after
15 minutes, the maximum reading should be 0.4%. If not, the sensor is no
longer zero current free and must be cleaned or sent to the manufacturer
for tests. After the test, the sensor should be rinsed thoroughly with
distilled water to remove any remaining traces of sodium sulfite solution.
Galvanic
sensors with lead counter may be submersed for no more than 3 minutes.
Subsequently, they must also be rinsed thoroughly with distilled water.
Cleaning of the sensors is extremely important to prevent toxification and
lasting damage.

Measurement
and analytical quality assurance:
Measurement
of the oxygen concentration is now quite easy to carry out. The sensor is
submersed in the liquid to be investigated and the measured value is read
from the display. In principle, this is all there is to it, but
nevertheless a few important points should be observed and among those is
the proper maintenance of the sensors.

Cleaning
of sensors:
The
component of the sensor that is sensitive to contamination is the
membrane. Contamination results in lower readings when measuring or lesser
slopes when calibrating because a portion of the membrane surface is not
available for the diffusion of oxygen. The attempt to compensate for the
contamination by adjusting the instrument does not agree with the water
principle. It is preferable to clean the membrane. Acetic or citric acid
with a concentration of 5--10% (percent in weight!) is used for calcium
and iron oxide deposits and warm (<50C) household detergent is used for
fats and oils.
Avoid
strong mechanical treatment of the membrane during all cleaning activities
because its thickness is on the order of m and it is easily destroyed. It
is best to use a soft paper towel. Dissolved oxygen not clean the sensor in an
ultrasound bath as this may cause the coating of the anodes to peel off.

Regeneration
of sensors:
Regeneration
of the sensor becomes necessary when the function responds or when the
slope (S) < 0.6 has decreased markedly when calibrating.
Basically,
regeneration is required when the electrolyte solution is depleted, when
the gold cathode has become coated with lead or silver, when the reference
electrode is to xified or when the membrane is damaged or contaminated.
It
consists of exchanging the electrolyte solution, cleaning the electrodes
and exchanging the membrane head.
It
is important to follow the operating manual exactly! Mistakes are then
easily avoided.
The
following points should be emphasized:
-
The
sensor must be disconnected from the meter. When the sensor is
connected and submersed in the cleaning solution, no chemical reaction
takes place between the solution and the oxidized reference electrode
surface; instead, the cleaning solution may become electrolyzed!
-
Use
the cleaning or electrolyte solution suitable for the particular
sensor as stated in the operating manual! A solution that is suitable
for silver electrodes cannot regenerate lead electrodes!
-
Only
the gold cathode should be polished; the counter electrode is merely
wiped clean with a soft cloth to wipe away easily removable salt
deposits! A spotty coating after regeneration of the lead or silver
electrodes does not impair measurements!
-
When
polishing the gold electrode, only use the moistened EID abrasive film
that has a special grain that polishes and do not scratch!
-
It
is also recommended to use a new membrane head since the used membrane
cannot necessarily guarantee that the membrane fits correctly against
the gold cathode which is ensured by a spacing lattice on the inside
of the membrane. Baggy clothing don't fit either!
Please
note: The spacing lattice is clearly visible when the membrane head
is held up against the light.
The
result of an oxygen measurement can be documented in several ways:
-
Display
of the concentration: The instrument requires the appropriate data of
the calibration curve and uses them to calculate the concentration in
mg/L (ppm is identical in this case), allowing for the temperature
dependency of the individual parameters
-
Display
of the percentage of oxygen saturation: The instrument measures the
sensor current and calculates the partial pressure of oxygen according
to the calibration. The current air pressure is measured for the
calculation of the saturation partial pressure. The display
corresponds to the quotient, converted into a percentage.

Polarization
periods (startup periods) prior to measurement:
If
the sensor was disconnected from the meter, an appropriate polarization
period must elapse after the polarographic sensors are reconnected
(gold-silver electrode system) before the start of measurements. Please be
advised that this does not apply to galvanic sensors (gold-lead electrode
system) because they are self polarizing and can be used immediately.

Approach
flow:
The
approach flow to the sensor membrane must be continuous for oxygen
measurements to be correct. The diffusion of the oxygen molecules in the
sensor head creates an oxygen poor zone that simulates a reduced
concentration of oxygen. The concentration at the membrane must always be
the equal to the concentration in the remainder of the sample. This
condition can be met by stirring the sample or moving the sensor in the
sample.
EID
offers special stirring attachments that rotate like little turbine blades
and continuously supply the membrane with fresh sample. They are driven by
an alternating electromagnetic field that is generated by the base of the
stirrer.
The
major advantage of this unit is the size of the attachment. It has the
same diameter as the sensor and is mounted on the sensor head. This
simplifies measuring in sample bottles such as bottles for BOD
measurements.
The
EID-E-ADO–A001 sensor is specially designed for BOD measurements. The
sensor shaft contains a propeller similar to a marine screw propeller to
maintain a continuous approach flow at the membrane. The stirring effect
is sufficiently large to homogenize the sample in addition to generating
the approach flow.
If
agitators or magnetic stirrers are used, the possible formation of eddies
must be taken into account. The oxygen sensor may not be positioned in the
eddy because air at the sensor head
Approach
flow membrane may falsify readings. This can be prevented by lowering the
stirring frequency or positioning the sensor away from the eddy.
When
the sensor is installed in pipelines, the sample flows past the sensor
head, providing a sufficient approach flow. EID offers stationary
measuring systems with special installation assemblies for pipes.
Alternatively,
the sensor itself can be moved in the medium being investigated, e.g. by
stirring the sensor in a beaker or by swinging it back and forth in a
lake. For measurements at great depths, depth armatures for water depths
up to 100m are available.
It
is important that stirring does not falsify the measured values. This is
likely to happen when the investigated sample is supersaturated or under
saturated with oxygen and oxygen can be expelled from or stirred into the
sample.
Super
saturation with oxygen, for example, can be observed in the summer in
stagnant waters when luxuriating algae produce oxygen by photosynthesis.
An example of under saturation with oxygen is the BOD determination in
which bacteria lower the oxygen concentration in bottles through
respiration. For this reason, the volume of the sample is important. A
measurement taken in a lake or an activation basin is uncritical because
of the enormous quantity of the sample. In an open beaker, however,
stirring can easily alter the oxygen concentration.

Correction
for salt content:
The
temperature dependent Bunsen absorption coefficient changes when
substances are dissolved in the water. This effect is accounted for by
entering the salinity. The salinity can be determined using a conductivity
meter and corresponds to the salt content of seawater in g/kg. The
standard recommends the use of this function for other waters since the
deviation is minimal (<2%).

Influence
of interfering gases:
The
membrane is also permeable to gases other than oxygen. Nitrogen does not
react and is, therefore, irrelevant. The high pH value of the electrolyte
solution protects the measurement from the interfering influence of
ammonia. Carbon dioxide, on the other hand, is problematic. The buffering
capability of the electrolyte solution is sufficient for short-term
exposure; during long-term exposure, however, carbon dioxide shifts the pH
value into the acidic range and leads to increased values. Polarographic
sensors can better regenerate the buffer capability than galvanic sensors
because they generate an excessive number of hydroxide ions during the
electrode reactions. The buffer capacity of the electrolyte solution in
the sensor is insufficient for samples with a high carbon dioxide content
(e.g. beer, sparkling wine or soft drinks). The pH shifts into the acidic
range and the meter shows higher than normal readings. Hydrogen sulfide
presents the greatest danger for oxygen sensors because the sulfide-ions
generated by the neutralization reaction toxify the counter electrode. The
sensors can withstand small amounts, but continuous exposure markedly
shortens their lifetime. Hydrogen
sulfide has the smell of rotten eggs and is easily perceptible at very
small concentrations, eliminating the need for complex measurements.

Solubility
functions:
In
order to determine the concentration of dissolved oxygen in non-aqueous
liquids, the appropriate solubility function must be known. High
performance dissolved oxygen meters and logger from EID have stored software programs
that make this type of determination feasible. If the solubility function
is known, oxygen measurements can be carried out similarly to measurements
in water.

Checking
the oxygen meter and or logger:
The
METER-D0 (meter) or
PROBER-DO (data logger) are checked using simulators.
The simulators are connected to the instrument in place of the sensor.
They generate defined current signals that the instrument must display
correctly. If the readings lie outside the tolerance indicated by the
certificate, the instrument must be sent to the manufacturer for
servicing.

Applications:
A.
Foods and Beverages
Many foodstuffs are packed in a Modified Atmosphere Packaging where a low
or controlled oxygen level is necessary. Dissolved oxygen levels in some
drinks, such as beer, should be kept in specific range. Practice of adding
oxygen under pressure to bottled water to make oxygenated water has become
more common. These dissolved oxygen measurements required dissolved oxygen
probes that can be cleaned at elevated temperatures without being removed
from the application.
B. Environmental monitoring
EID's dissolved oxygen data loggers can be left to record dissolved oxygen
fluctuations in lakes, rivers etc. Deep sea oxygen probes are used in
oceans and deep lakes. EId's dissolved oxygen electrodes with fast
response are used to map the dissolved oxygen content of lakes and fishing
waters. EId's dissolved oxygen probes are not only raised and lowered in
the water, but also towed through the water at different depths to give a
total picture of the state of the area concerned.
C.
Fish Farming - Aquaculture
Fish farmers needed multi-channel dissolved oxygen meters. Additionally,
they need dissolved oxygen monitoring and dissolved oxygen control
equipment. EID's dissolved oxygen monitoring and logger are encompass
alert units with both high dissolved oxygen alarm and low dissolved oxygen
alarm. Equipment introduced by EID in 1977 for controlling dissolved oxygen
they are been used all over the world.
D. Water treatment (Re-circulating)
The water is cleaned and filtered through mechanical and biological
filters. Ozone can be added to "burn off" pollutants, either by
direct ozone injection or by UV ozone activation. This process can be
controlled using a redox or ORP measurement. The pH of the water is
measured and controlled using a
pH meter and pH
controller. The dissolved oxygen content is measured and pure oxygen is
injected. This oxygen injection can also be used to strip off carbon
dioxide. Often only a small proportion of the water is oxygenated at high
pressure. The resulting super-saturated water is mixed with the main flow
to give healthy dissolved oxygen levels in the growth tanks. EID's
In-line
dissolved oxygen electrodes,
Twist
and lock mount dissolved oxygen electrodes or flow cell dissolved oxygen
electrodes can be used in such high pressure oxygenation systems.
E. Hatchery and growth tanks
Water level as well as dissolved oxygen should be measured in each tank -
the water supply to one tank could be cut off. Oxygen level alarms are set
on the dissolved oxygen measurements. Aeration or oxygen injection to each
tank is not often practiced in smaller indoor tanks, oxygen is added to
the inlet or re-circulated water. Aeration or oxygen injection is,
however, seen in larger tanks, requiring a separate dissolved oxygen meter
with dissolved oxygen controller system for each tank. This is easily done
with EID's MultiProbe TechnologyTM dissolved oxygen metering,
logging and control equipment.
F. Sea cages
Since it is difficult to control the dissolved oxygen content of the sea.
Dissolved oxygen measurement is very important because feed uptake and dissolved oxygen
levels are interconnected. Intensive feeding after fish have experienced
low dissolved oxygen levels can not only be a waste of food, but can
actually harm the fish. The measurement of dissolved oxygen levels enables
feed to be dosed optimally and, if relayed to the shore can warn that the
cage should be moved if extremely low dissolved oxygen levels should
occur.
G. Transport tanks
Dissolved oxygen measurement should also be performed during transport to
the processing plant. A healthy fish gives a better finished product when
contain the right level of oxygen. Another situation requiring dissolved oxygen
measurement is the transport of juvenile fish to tanks or cages for
growing.
H. Oxygen
generator control
Pure oxygen meters and oxygen controllers equipment are also used in
aquaculture. The purchase of liquid oxygen in bulk is often the most
economic solution, but there are many cases where oxygen generators are
installed locally. Two of the many advantages of using pure oxygen are
that 1) it is possible to super-saturate the water with oxygen and 2) you
save pump energy since pumping air means pumping 79% nitrogen and
"only" 20.9% oxygen.
I. Waste Water Treatment
It is no longer enough just to filter the water and dump the detritus in
the sea. The larger part of the waste is mainly organic, and this must be
broken down in sludge tanks and the effluent water controlled and treated
as necessary.
Sludge tank dissolved oxygen measurement and control is kept at
approximately 2 mg/l.
Flow measurement, suspended solids measurement, sludge blanket detection,
conductivity
measurement,
nitrate measurement
and phosphate measurement utilizing
EID's
Industrial electrodes are also all used to enable the efficient and
effective cleaning of waste water.
J. Safety
Monitoring
Both oxygen detection in flammable gas and oxygen monitoring in ambient
air are examples of this. Blanket gas is often used where flammable
substances occur. Blanket gas is gas that cannot burn or sustain fire,
i.e. it does not contain oxygen. Volumetric oxygen measurement is carried
out both on the blanket gas and the surrounding air, the latter for worker
safety. Special versions of the EID's dissolved oxygen electrodes are
approved for use in potentially dangerous atmospheres, i.e. in classified
areas.
K.
Measuring biochemical oxygen demand
The
BOD test requires a commitment of five (5) days from initial sample
collection to the end of the analysis. During this time, samples are
initially seeded with microorganisms and supplied with a carbon nutrient
source of glucose-glutamic acid. The sample is then introduced to an
environment suitable for bacterial growth at reproducible temperatures,
nutrient sources and light within a 20C incubator such that oxygen will be
consumed. Quality controls, standards and dilutions are also run for
accuracy and precision. Determination of the dissolved oxygen within the
samples can be determined through titration. The difference in initial DO
readings (prior to incubation) and final DO readings (after a five (5) day
incubation period) predicts the BOD of the sample. A suitable detection
limit as per environmental quality control is 1 mg/l.
Steps
to calculate Biochemical Oxygen
Demand (BOD). They and are based on the addition of a nutrient source
(carbon - glucose - glutamic acid) and no nutrient source.
1.
The BOD of the blanks (no nutrient source) = DOFinal - DOInitial
2.
The BOD of the nutrient added samples = (DOFinal - DOInitial)
time dilution factor per 300ml
*
300 ml is based on the volume contained in BOD bottles
The
BOD of the sample and standards are calculated by subtracting the final DO
from the initial DO and multiplying this factor by the dilution factor.
The final value is determined by subtracting out the BOD for the blank
from the BOD that has been nutrient enriched.

Practical
experiments:
Preparations:
All
practical experiments should be carried out in a suitable laboratory to
guarantee working safety. This is a general recommendation. In the field
of oxygen measurement, the cleaning solutions and the electrolyte
solutions may contain caustic substances. A possible danger therefore
exists when regenerating the sensor.
Safety
instructions. General rules of conduct when handling chemical substances
When
working at a work place at which chemicals are handled, the following
rules must be observed:
1.
Follow the instructions on the chemical bottles
2.
Always wear protective garments (goggles, gloves...)
3.
Never point open containers towards other persons
4.
Do not eat, drink or smoke
5.
Ensure the satisfactory disposal of chemicals
6.
Carefully remove or clean up any spilled chemicals
7.
Contact specialist personnel if any serious problems arise
We
provide these short instructions in the hope that they lead to successful
and safe practical research and studies.
Safety
data sheets are available for the cleaning and electrolyte solutions. The
user must have one of these data sheets. Because they are fairly
comprehensive, they cannot be included in every delivery. The manufacturer
will, however, provide them on request.
The
following equipment and facilities must be present when you are measuring
dissolved oxygen:
|